THE END OF A FAIRY TALE: THE RISE OF CRISPR FROM RARE HOPE TO REAL THERAPY
By: Aly Diana
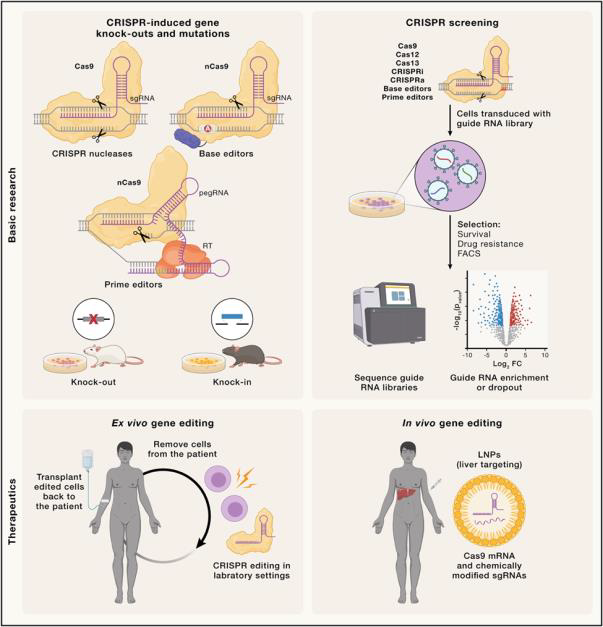
A while ago, I discussed CRISPR (Clustered Regularly Interspaced Short Palindromic Repeats)—and honestly, the idea of editing genes still fascinates me. I remain deeply hopeful about what it could mean for the future of medicine. From time to time, I check on its progress, and I was delighted (even if a little late!) to learn that a CRISPR/Cas9‑based therapy—exagamglogene autotemcel, or exa‑cel—was approved in the United Kingdom in November 2023 and by the U.S. Food and Drug Administration in December 2023. It was cleared as a treatment for sickle cell disease (SCD) and transfusion-dependent β‑thalassemia (TDT). This milestone feels like a powerful leap forward: it proves that CRISPR is no longer a fairy tale—it is here, and it works. It also renews hope for patients facing other genetic and even infectious diseases.
Exa‑cel has already shown impressive efficacy in clinical trials. In a Phase 3 study of patients with severe SCD, 97 percent (29 of 30) were free from severe vaso‑occlusive crises for at least 12 consecutive months after treatment. Among individuals with TDT, 91 percent (32 of 35) achieved transfusion independence following exa‑cel therapy. The safety profile of exa‑cel was generally favorable, and no deaths or cancers occurred. After treatment, participants are invited to enroll in a 13‑year follow‑up study (CLIMB‑131) to monitor the durability and safety of this gene‑editing approach over time.
CRISPR technologies are evolving rapidly. The discovery of Cas9 sparked a wave of research that uncovered other RNA‑guided enzymes suitable for genome editing. Among these, Cas12a stands out for its distinct target‑recognition pattern and generally higher precision. Scientists have since identified a range of smaller, more specialized nucleases—such as Cas12g and Cas13—that offer advantages in delivery and RNA editing. These newer enzymes also enable applications such as mRNA editing and diagnostic tools. Although each enzyme has its own strengths and limitations—such as target restrictions or potential immunogenicity, the expanding toolkit allows greater flexibility and precision in gene editing.
CRISPR has revolutionized biomedical research by enabling precise disease modeling, large‑scale genetic screening, and even biological recording systems. In diagnostics, it is used for fast, sensitive detection of pathogens. In therapy, gene editing has progressed from preclinical models to clinical trials, employing both in vivo and ex vivo approaches. Ex vivo editing has advanced particularly far in cancer treatment through genetically modified immune cells. A recent review in Cells (2024) is highly recommended for a deeper understanding—it is an engaging read.
In infectious‑disease research, one especially interesting focus is HIV. CRISPR has been studied against HIV for more than a decade. The first demonstration of CRISPR/Cas9 technology applied to HIV‑1/AIDS treatment appeared in 2013, when researchers suppressed HIV‑1 gene expression in Jurkat cell lines by targeting two critical regions within the HIV‑1 long terminal repeat: the NF‑κB binding motifs in the U3 region and the TAR sequences within the R region. Many studies have followed. One of the most recent and promising experiments involved HIV‑1 ADA‑infected CD34⁺ NSG‑humanized mice. Treatment with long‑acting ester prodrugs of cabotegravir, lamivudine, and abacavir, combined with native rilpivirine, was followed by dual CRISPR/Cas9 gene editing that targeted CCR5 and HIV‑1 proviral DNA. This sequential approach achieved viral suppression, restored absolute human CD4⁺ T‑cell counts, and eliminated replication‑competent virus in 58 percent of the infected mice. Dual CRISPR therapy facilitated the excision of integrated proviral DNA in infected human cells within live animals. These findings highlight the diverse potential targets within the HIV‑1 genome that could be exploited to reverse latency in infected cells. However, further research is essential to identify the most effective genomic targets and to optimize the therapeutic use of CRISPR/Cas9 technology in HIV‑1 treatment.
Although using CRISPR to defeat HIV will require more time, the approval of exa‑cel shows what is possible. Personally, it is hard not to feel hopeful. Seeing CRISPR move from theory to therapy feels like living in an era of real scientific magic. And although CRISPR‑based treatments for infectious diseases such as HIV are not here yet, they are closer than ever.
References
- Borrajo A. Breaking barriers to an HIV‑1 cure. Life (Basel) 2025;15:276. doi:10.3390/life15020276.
- Frangoul H et al.; CLIMB SCD‑121 Study Group. Ex-agerologene autotemcel for sickle‑cell disease. N Engl J Med 2024;390:1649‑1662. doi:10.1056/NEJMoa2309676.
- Hussein M et al. CRISPR‑Cas cure for HIV/AIDS. Int J Mol Sci 2023;24:1563. doi:10.3390/ijms24021563.
- Locatelli F et al.; CLIMB THAL‑111 Study Group. Ex-agamglogene autotemcel for β‑thalassemia. N Engl J Med 2024;390:1663‑1676. doi:10.1056/NEJMoa2309673.
- Pacesa M, Pelea O, Jinek M. CRISPR genome‑editing technologies. Cell 2024;187:1076‑1100. doi:10.1016/j.cell.2024.01.042.
- Lancet Haematology. Dawn of the CRISPR/Cas9 gene‑therapy era. Lancet Haematol 2024;11:e1. doi:10.1016/S2352‑3026(23)00372‑1.
Most Commented